Introduction
I have decades of experience participating in vigorous outdoor activities that cause the same result: sweat-soaked base layers, wet skin, and resulting discomfort. I expect others have had similar experiences. There have been many discussions at Backpacking Light on proper layering techniques to counter this problem (see this forum thread as an example).
I find many of these techniques are often unsuccessful.
People don’t always understand how wicking layers function and how use conditions in a multilayer and even a single layer ensemble can degrade the ability of an otherwise capable fabric to move moisture from the skin to the environment.
This article will explore the factors that prevent wicking fabrics from performing their intended function.
I am going to describe general use scenarios that degrade wicking fabric function. I will provide test results that demonstrate the impacts of these general use scenarios. My goal is to provide outdoor adventurers with an understanding of why their wicking base layers may fail. This work will provide a basis for future articles in which I will discuss how to avoid the pitfalls of common layering approaches.
I will discuss the following topics in this article:
1. The most critical element of a wicking fabric is how well it allows sweat to be drawn away from the skin, converted to moisture vapor, and then transferred out of any clothing layers. This element is different from how well the fabric itself wicks. How well the fabric wicks does not determine whether you will be comfortable. The disposition of sweat to the environment depends on much more than whether a wicking fabric can absorb lots of moisture in a laboratory test.
2. Standard wicking tests usually measure how much moisture fabric absorbs (and how fast this happens). This data may be useful information but is inadequate to help the user understand what kind of performance to expect, even when the base layer performs very well in laboratory tests.
3. Wicking performance and drying rate of wicked moisture are related. But these two necessary components for wearer comfort are driven by unrelated physical processes. The best wicking fabric won’t keep you dry if the drying conditions limit evaporation. Similarly, if drying conditions are optimal, a wicking fabric may not have the capacity to keep up, resulting in possible user discomfort.
4. When your wicking layer is your outer layer, increased airspeed will substantially improve drying.
5. When your wicking layer is your outer layer, increased relative humidity will substantially degrade drying.
6. If your wicking base layer is in a multiple-layer system, drying is restricted by limited airflow and high relative humidity on the outside of the garment. The garment can reach saturation, at which point, sweat will drip out of the wicking fabric and go wherever gravity dictates.
Before plunging ahead, you may want to review the fundamentals of wicking in my last article. These concepts are important for understanding the information provided below.
About this Series
By the Numbers is a column by Backpacking Light contributor Stephen Seeber, who turns a critical eye towards fabrics and materials by testing for claims, degradation, and more.
What is wicking?
There is a vast array of literature available to describe and measure wicking. Most of it focuses on:
- How much moisture can move into a piece of fabric?
- How high can the moisture move?
- How fast can it spread?
Much of the research and most standard tests occur in a “standard environment” of 68 °F (20 °C) and 65% relative humidity (RH). Sometimes, testers apply low-level airflow over a test sample. Sometimes, testers use still air. Research and standards typically examine a single fabric, not an ensemble. These scenarios are very different from real-life use, where wind and humidity can vary widely, and a wicking fabric may be a base layer in an ensemble or the only worn layer.
There is an expansive marketing machine that talks about keeping you dry. However, is dry referring to the skin surface, the wicking layer, or the outer layers? Who knows? However, this seemingly limitless marketing machine has been delivering its message that a wicking layer next to the skin is critically important to comfort. We have grown to accept this even in the face of repeated outings that result in both wet skin and a wet wicking base layer.
In my opinion, both miss the reality of what we want our clothing choices to accomplish. I have a different goal: I want to get moisture away from my body and into the environment. My objective is dry skin and dry layers. This objective will keep us comfortable. Is a wicking layer the best way to achieve this objective? Perhaps not.
Here is the disconnect between real-life use, research/test standards, and the manufacturer’s claims: it does not matter how fast wetting starts or how much capillary capacity is present if the moisture stays in the wicking fabric.
The problem is that the absorbed and transported moisture has to dry. To dry, moisture must evaporate and then transfer through all layers to reach the environment. The drying rate of the wicking fabric somehow must match the wicking rate, and the wicking rate must match the rate at which the wearer sweats. If the drying rate does not match the wicking rate, the wetting rate, and the sweating rate, you end up with a wet wicking layer.
Unfortunately, the drying process occurs independently of the wicking process. There is no physical reason that the drying rate must match the wicking rate. It might, but there are many reasons it won’t. The physical drivers of wicking and drying are independent of one another.
Fundamentally, the design of the wicking fabric can significantly influence wicking performance. However, it may not have much impact on how fast drying occurs. That mismatch is a recipe for a wet wicking layer.
Article Library Resources: Read more Backpacking Light articles about wicking and moisture management:
How do fabrics dry?
Liquid water consists of numerous water molecules attracted to one another by cohesive forces. These molecules are in constant motion. The average rate of motion reflects the water temperature. The rate of motion for individual molecules in water will range from high to low. The distribution of the rate of motion is not uniform. Some molecules will have much higher energy levels and exhibit a higher rate of motion. When the motion of molecules exceeds a certain velocity, the energy available exceeds the cohesive force of surrounding water molecules, and the high motion/high energy molecules can escape to the atmosphere.
This process of escape is called evaporation. Evaporation occurs at the water surface (as opposed to boiling water, where molecules can escape from beneath the surface). Evaporation does not require temperatures at or above boiling, as is clear from watching a puddle disappear from the pavement during the winter.
The rate of evaporation increases by several means:
- As air pressure decreases, water molecules require less energy to escape (that is why water has a reduced boiling point at higher elevations).
- As water temperature increases, average molecular motion will increase, and more molecules will escape to the atmosphere.
- As relative humidity of the air decreases, the air can contain more water molecules, so less energy is required to support evaporation.
- As evaporation occurs, the relative humidity of the air in contact with the water surface will increase. These factors will slow evaporation. However, if the air is in motion and the relative humidity of the air flowing across the water surface is less than 100%, relative humidity at the water’s surface will remain low enough so that evaporation can continue – this is why water evaporates more rapidly in a breeze.
Generally, we cannot control the air pressure to regulate evaporation rate. However, we can readily impact the evaporation rate if we influence temperature levels or airflow for our layers or reduce relative humidity. Wicking layers cannot accomplish this. It requires a systematic approach to layering that recognizes all of these methods of promoting drying.
Our bodies typically provide heat to support evaporation from our wicking layers. If we wear wicking layers as an outer layer, the ambient air can provide additional energy to support drying. Airflow rate over a wicking layer’s exterior surface and the relative humidity of the air adjacent to the wicking layer both influence the wicking-layer drying rate. These are critical ingredients that the user must recognize to achieve drying and deliver moisture to the environment.
The impact of wind and relative humidity or drying performance
Let’s look at the results of a simple experiment to demonstrate the impact of both air velocity and relative humidity on evaporation from a wicking fabric. We conducted this experiment using our permeation kettles to perform a wick/dry test. This test is described in detail here. In this test, we measure the amounts of water wicked into a fabric, absorbed into the fabric, and evaporated from the fabric in still air and moving air (3 mph air velocity) at 25% and 65% relative humidity. The results are in Figure 1.
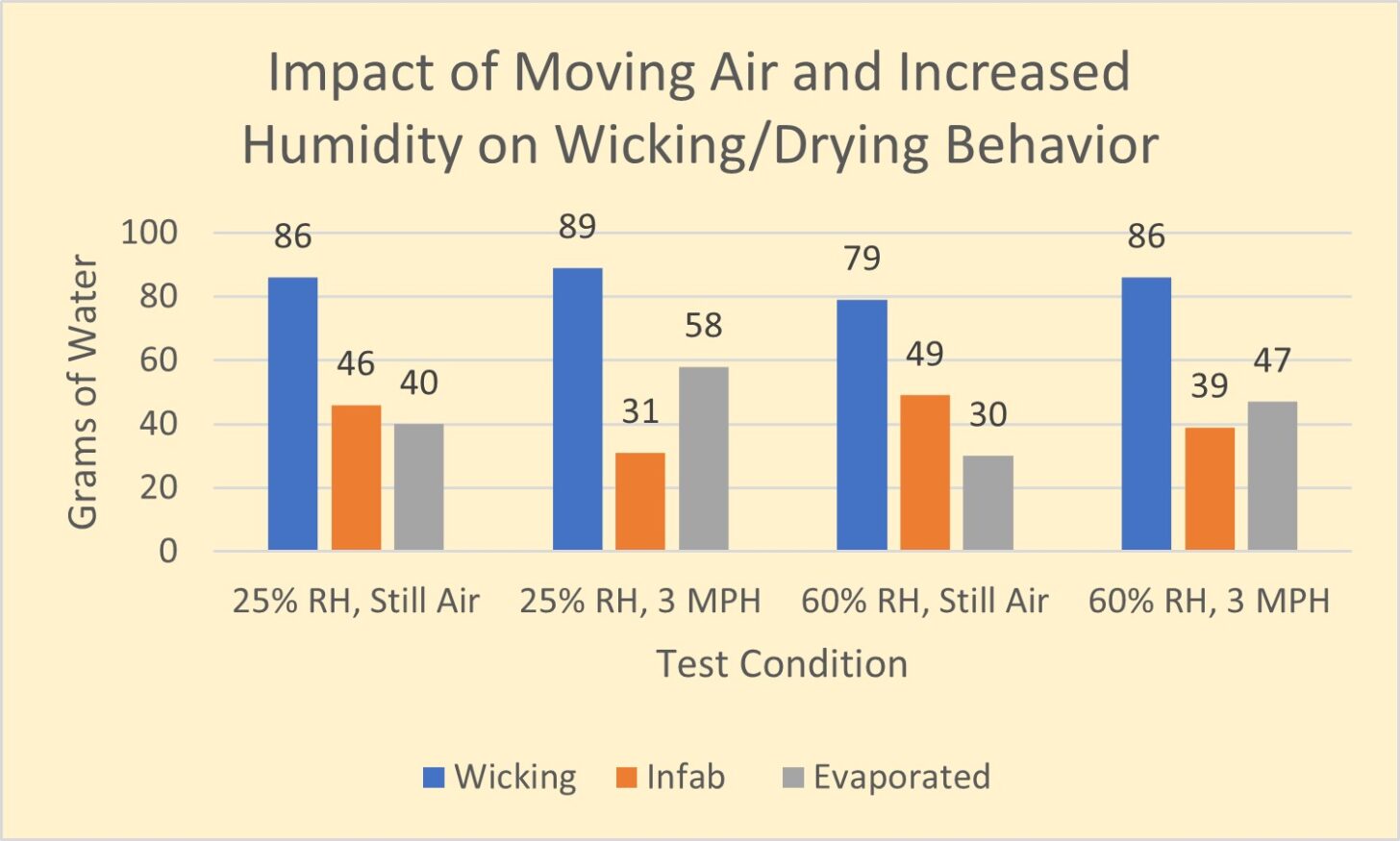
We will use several graphs in this format, so first, let’s explain the graph. This graph presents how much moisture enters a fabric and what happens to it afterward.
During the test, water transfers from a sponge into the test fabric. We termed the amount of water transferred into the test fabric during the test Wicking (blue vertical bar). We determine the amount of Wicking by weighing the sponge before and after the test. The weight difference is the quantity of water, in grams, that has been wicked into the fabric.
At the end of the test, the fabric will hold a certain amount of water. This quantity is Infab (orange vertical bar) on the graph. Infab means “water in the fabric.” The difference in fabric weight before and after the test determines the amount of water in the wicking fabric at the test’s end. Why is Infab important? The more water trapped in your wicking fabric, the more body heat you’ll expend attempting to dry the fabric when you stop moving. This energy expenditure can lead to chills and, if enough water is present, hypothermia. Infab can help you understand how miserable you might be once you stop generating excess heat.
Evaporation (gray bar) is the difference between Wicking and Infab. Evaporation is the amount of water that wicked into the test fabric but is not present in the fabric at the end of the test. Evaporation tells us how effectively the wicking fabric is performing.
In Figure 1, we see that with relative humidity at 25% in still air, 86 grams of water wicked into the fabric, 46 grams of water remained in the fabric at the end of the test and 40 grams of water evaporated. 47% of the wicked water evaporated during the test.
In the next set of vertical bars, we repeat the test at the same relative humidity but provide a 3 mph breeze. We can see that wicking increased, the “Infab” water reduced by 33%, and the evaporation increased by 45%.
We can readily see how increased airflow can profoundly impact the drying rate.
In the next set of vertical bars, we can see the impact of increasing the relative humidity from 25% to 60% in still air. We see that wicking has reduced. The amount of water – Infab – has increased, and evaporation has decreased from 40 to 30 grams: a 25% decrease in drying! Of course, if we increased the relative humidity to 100%, no evaporation would have occurred.
In the final set of vertical bars, we measure the impact of both elevated relative humidity and increased airflow. The wicking quantity returns to the level for still air and low humidity. However, both Infab and Evaporation performance is degraded compared to the low humidity, 3-mph airflow case.
It is clear from this simple example that increased airflow improves drying, and increased relative humidity degrades drying. This outcome is independent of fabric structure, fiber type, or chemical treatment of wicking fabrics.
The role of moisture spread and fabric thickness in drying performance
In popular discussions of wicking and academic research, reference is often made to the “spreading of moisture across the fabric to improve evaporation.” Spread rate is also an important metric and a surrogate for drying speed in some standardized tests. That is an appealing concept. We know that evaporation occurs on the water’s surface, so spreading the water will increase the surface area and improve the drying rate.
You don’t sweat a single drop at a single spot. You sweat from many pores in close proximity, and sweat can occur continuously. When one spreading drop encounters another, the spread stops at the contact area. Spreading can continue where the drops are not in contact. In the field, there is probably little surface area where drops are not in contact. We illustrate the impact of spread and contact in the video below. The video compresses about 7 minutes of drying time into 30 seconds.
For this demonstration, I apply four 50 uL drops of water to the surface of a permeation kettle. Two (on the right) are close together so that the moisture from one will contact the other as wicked water spreads. I place the other two drops where they can spread without encountering moisture.
The viewer can see the placement of the drops on the kettle surface in the video screenshot before the video starts. Immediately after the video begins, I place a wicking fabric over the drops. In real-time, moisture spreading reaches maximum size in 38 seconds. Spread stops when all the water applied to the kettle surface transfers into the fabric. The white ellipses around the drops indicate the size achieved by spreading. The drops are cool relative to surrounding surfaces due to evaporative cooling. The drops eventually diminish in size and increase in temperature until they disappear. At this point, they are dry. The size of the wet areas does not diminish until the 320-second mark. The two adjacent drops make contact in 12 seconds and reach their final size at 28 seconds. The combined surface area of the two separate drops is 722 pixels. The combined area of two adjacent drops is 586 pixels.
This observation demonstrates that spread does not maximize surface area when there are numerous close water sources. In fact, the two close drops are the last to disappear as drying occurs. I suggest that spread rate does not provide a useful surrogate for drying behavior. In real life, significant spread may not occur.
When adjacent wetting restricts moisture spread, the water volume in the thickness of the fabric increases over what it would be if spreading could occur. Evaporation (drying) occurs from the top surface of water. As evaporation proceeds, the volume of underlying water reduces as water molecules move to the surface and replace evaporated molecules. Drying appears to occur at the edges (see the video, seconds 19 to 30). This appearance of drying at the edges is probably an illusion. As the drop area loses water, the cohesive force between remaining water molecules of water causes the drop size to shrink and forces the drop to center itself over the area of greatest water volume in the thickness of the fabric. In the video, I show a plus sign (“+”) to mark the final position of the drop when it disappears.
Since the two drops on the right coalesced and had less spread than the other drops, their associated moisture volume in the underlying fabric pores increased compared to the stand-alone drops on the left. As a result, they were slower to dry. We can call this process anti-spread. As drying proceeds, the surface area diminishes, and drying occurs at a constantly slowing rate! The volume of water beneath the wet surface will strongly influence the time required to dry.
We can see that the rate of spread provides some useful information about wicking performance. However, it does not necessarily predict drying time. Spread does not reflect the volume of water associated with wicking. Suppose we have a thin and thick fabric with equal spread rates. The thin fabric will dry faster because there is less volume of water beneath the wet surface area. As we will see later, the drying rate (e.g., grams per minute) may well be the same for the light and heavy fabric and not particularly related to fabric properties. Fabric thickness and weight can both be good predictors of drying time. I’ll discuss the role of fabric thickness or density in greater detail in the next section.
What factors determine drying time?
To understand the critical factors that determine the drying time of a wicking fabric, we measured the drying times and drying rates of four different fabrics. These fabrics vary in weight, thickness, chemical treatment, and fiber composition. I only tested four fabrics, so this is not an exhaustive look at the possible range of variables. I conducted this test using saturated samples. I soaked the samples in warm water, then wrung out each sample until the fabric no longer produced water drops. The samples for a particular sample type were saturated to achieve nearly identical weight. I placed the samples on the permeation kettles and ran the wick/dry test in the usual manner for 30 minutes. I added this step to force more water into the fabric and achieve maximum saturation. Water forced into the fabric beyond saturation point would drip from the edges of the fabric where I captured it in paper towels so this water, like all water introduced into the test, could be accounted for. At the end of the test, the samples were removed and weighed. This step established how much water the fabric absorbed. I then placed the samples directly on the hot permeation kettle surfaces and allowed them to dry. Dryness was determined by plotting the average surface temperatures using the thermal imager. When the sample surface temperatures eventually increased and achieved their maximum temperatures, the fabrics were dry, and the test ended. This procedure provides drying time and calculation of the drying rate in grams per minute.
Member Exclusive
A Premium or Unlimited Membership* is required to view the rest of this article.
* A Basic Membership is required to view Member Q&A events
Home › Forums › Why is my base layer soaked?